The nervous system is like a highly adaptable orchestra, constantly fine-tuning its performance for optimal function. To achieve this, it employs a variety of mechanisms, including homeostatic plasticity—the ability to make stabilizing adjustments. A recent discovery in the field of neuroscience is the axon initial segment (AIS), a microdomain at the start of the axon that can undergo structural changes in response to activity perturbations. However, much about AIS plasticity and its role in regulating neuronal excitability remains unclear due to its complex nature. To tackle this challenge, researchers have turned to high-density microelectrode arrays (HD-MEAs) as a powerful tool to track changes in AISs over time. By analyzing extracellular electrophysiological readouts, they aim to identify specific activity signatures that correspond to microstructural changes in the AIS. In this computational study, sophisticated models were employed to explore the relationship between AIS position changes and observed consequences in simulated extracellular field potentials. Remarkably accurate models were developed to detect characteristic features that signify AIS plasticity. These findings pave the way for a hybrid analysis framework and high-throughput experimental studies using HD-MEAs to unravel the mysteries of activity-dependent AIS plasticity.
Despite being composed of highly plastic neurons with extensive positive feedback, the nervous system maintains stable overall function. To keep activity within bounds, it relies on a set of negative feedback mechanisms that can induce stabilizing adjustments and that are collectively termed “homeostatic plasticity.” Recently, a highly excitable microdomain, located at the proximal end of the axon—the axon initial segment (AIS)—was found to exhibit structural modifications in response to activity perturbations. Though AIS plasticity appears to serve a homeostatic purpose, many aspects governing its expression and its functional role in regulating neuronal excitability remain elusive. A central challenge in studying the phenomenon is the rich heterogeneity of its expression (distal/proximal relocation, shortening, lengthening) and the variability of its functional role. A potential solution is to track AISs of a large number of neurons over time and attempt to induce structural plasticity in them. To this end, a promising approach is to use extracellular electrophysiological readouts to track a large number of neurons at high spatiotemporal resolution by means of high-density microelectrode arrays (HD-MEAs). However, an analysis framework that reliably identifies specific activity signatures that uniquely map on to underlying microstructural changes is missing. In this study, we assessed the feasibility of such a task and used the distal relocation of the AIS as an exemplary problem. We used sophisticated computational models to systematically explore the relationship between incremental changes in AIS positions and the specific consequences observed in simulated extracellular field potentials. An ensemble of feature changes in the extracellular fields that reliably characterize AIS plasticity was identified. We trained models that could detect these signatures with remarkable accuracy. Based on these findings, we propose a hybrid analysis framework that could potentially enable high-throughput experimental studies of activity-dependent AIS plasticity using HD-MEAs.
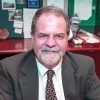
Dr. David Lowemann, M.Sc, Ph.D., is a co-founder of the Institute for the Future of Human Potential, where he leads the charge in pioneering Self-Enhancement Science for the Success of Society. With a keen interest in exploring the untapped potential of the human mind, Dr. Lowemann has dedicated his career to pushing the boundaries of human capabilities and understanding.
Armed with a Master of Science degree and a Ph.D. in his field, Dr. Lowemann has consistently been at the forefront of research and innovation, delving into ways to optimize human performance, cognition, and overall well-being. His work at the Institute revolves around a profound commitment to harnessing cutting-edge science and technology to help individuals lead more fulfilling and intelligent lives.
Dr. Lowemann’s influence extends to the educational platform BetterSmarter.me, where he shares his insights, findings, and personal development strategies with a broader audience. His ongoing mission is shaping the way we perceive and leverage the vast capacities of the human mind, offering invaluable contributions to society’s overall success and collective well-being.