Resting-state brain activities have been extensively investigated to understand the macro-scale network architecture of the human brain using non-invasive imaging methods such as fMRI, EEG, and MEG. Previous studies revealed a mechanistic origin of resting-state networks (RSNs) using the connectome dynamics modeling approach, where the neural mass dynamics model constrained by the structural connectivity is simulated to replicate the resting-state networks measured with fMRI and/or fast synchronization transitions with EEG/MEG. However, there is still little understanding of the relationship between the slow fluctuations measured with fMRI and the fast synchronization transitions with EEG/MEG. In this study, as a first step toward evaluating experimental evidence of resting state activity at two different time scales but in a unified way, we investigate connectome dynamics models that simultaneously explain resting-state functional connectivity (rsFC) and EEG microstates. Here, we introduce empirical rsFC and microstates as evaluation criteria of simulated neuronal dynamics obtained by the Larter-Breakspear model in one cortical region connected with those in other cortical regions based on structural connectivity. We optimized the global coupling strength and the local gain parameter (variance of the excitatory and inhibitory threshold) of the simulated neuronal dynamics by fitting both rsFC and microstate spatial patterns to those of experimental ones. As a result, we found that simulated neuronal dynamics in a narrow optimal parameter range simultaneously reproduced empirical rsFC and microstates. Two parameter groups had different inter-regional interdependence. One type of dynamics was synchronized across the whole brain region, and the other type was synchronized between brain regions with strong structural connectivity. In other words, both fast synchronization transitions and slow BOLD fluctuation changed based on structural connectivity in the two parameter groups. Empirical microstates were similar to simulated microstates in the two parameter groups. Thus, fast synchronization transitions correlated with slow BOLD fluctuation based on structural connectivity yielded characteristics of microstates. Our results demonstrate that a bottom-up approach, which extends the single neuronal dynamics model based on empirical observations into a neural mass dynamics model and integrates structural connectivity, effectively reveals both macroscopic fast, and slow resting-state network dynamics.
Read Full Article (External Site)
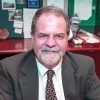
Dr. David Lowemann, M.Sc, Ph.D., is a co-founder of the Institute for the Future of Human Potential, where he leads the charge in pioneering Self-Enhancement Science for the Success of Society. With a keen interest in exploring the untapped potential of the human mind, Dr. Lowemann has dedicated his career to pushing the boundaries of human capabilities and understanding.
Armed with a Master of Science degree and a Ph.D. in his field, Dr. Lowemann has consistently been at the forefront of research and innovation, delving into ways to optimize human performance, cognition, and overall well-being. His work at the Institute revolves around a profound commitment to harnessing cutting-edge science and technology to help individuals lead more fulfilling and intelligent lives.
Dr. Lowemann’s influence extends to the educational platform BetterSmarter.me, where he shares his insights, findings, and personal development strategies with a broader audience. His ongoing mission is shaping the way we perceive and leverage the vast capacities of the human mind, offering invaluable contributions to society’s overall success and collective well-being.