
Cingulate Cortex Atrophy Is Associated With Hearing Loss in Presbycusis With Cochlear Amplifier Dysfunction
Chama Belkhiria1,2, Rodrigo C. Vergara1,2, Simón San Martín1,2, Alexis Leiva1,2, Bruno Marcenaro1,2, Melissa Martinez3, Carolina Delgado1,3 and Paul H. Delano1,2,4*
1Department of Neuroscience, Faculty of Medicine, University of Chile, Santiago, Chile
2Biomedical Neuroscience Institute, Faculty of Medicine, University of Chile, Santiago, Chile
3Department of Neurology and Neurosurgery, Clinical Hospital of the University of Chile, Santiago, Chile
4Department of Otolaryngology, Clinical Hospital of the University of Chile, Santiago, Chile
Age-related hearing loss is associated with cognitive decline and has been proposed as a risk factor for dementia. However, the mechanisms that relate hearing loss to cognitive decline remain elusive. Here, we propose that the impairment of the cochlear amplifier mechanism is associated with structural brain changes and cognitive impairment. Ninety-six subjects aged over 65 years old (63 female and 33 male) were evaluated using brain magnetic resonance imaging, neuropsychological and audiological assessments, including distortion product otoacoustic emissions as a measure of the cochlear amplifier function. All the analyses were adjusted by age, gender and education. The group with cochlear amplifier dysfunction showed greater brain atrophy in the cingulate cortex and in the parahippocampus. In addition, the atrophy of the cingulate cortex was associated with cognitive impairment in episodic and working memories and in language and visuoconstructive abilities. We conclude that the neural abnormalities observed in presbycusis subjects with cochlear amplifier dysfunction extend beyond core auditory network and are associated with cognitive decline in multiple domains. These results suggest that a cochlear amplifier dysfunction in presbycusis is an important mechanism relating hearing impairments to brain atrophy in the extended network of effortful hearing.
Introduction
Age-related hearing loss or presbycusis is characterized by bilateral progressive hearing loss and impaired speech understanding, especially in noisy environments (Gates and Mills, 2005). According to recent epidemiological data from the United States more than 50% of people aged over 70 years have presbycusis (Goman and Lin, 2016). In addition, due to population aging, it is expected that by the year 2025 there will be more than one billion people with hearing loss in the world (Sprinzl and Riechelmann, 2010). The increasing number of hearing loss patients is alarming, since epidemiological evidence has shown an association between presbycusis and cognitive decline in elderly people (Lin and Albert, 2014; Thomson et al., 2017). For instance, Lin et al. (2011) have shown that individuals with mild to moderate presbycusis have worse results in executive function and psychomotor processing, while other studies have shown that hearing loss is significantly related to global cognitive decline, which can lead to social isolation and depression (Harrison Bush et al., 2015; Panza et al., 2015). Furthermore, a recent prospective cohort has reported that presbycusis subjects with audiometric hearing thresholds worse than 40 dB are more likely to develop dementia (Deal et al., 2017). In line with this evidence, a Lancet consortium recently proposed a model in which hearing loss is the major potentially preventable risk factor for dementia (Livingston et al., 2017).
Although epidemiological and clinical studies confirm the relationship between hearing loss and cognitive decline, the mechanisms that relate them remain elusive (Lin and Albert, 2014; Wayne and Johnsrude, 2015). The pathological correlate of presbycusis can display different features, including cochlear hair cell loss, stria vascularis atrophy, and auditory-nerve neuron loss (Gates and Mills, 2005). Animal models of presbycusis have shown that in addition to the peripheral auditory damage, aged animals have less GABAergic neurons in the auditory cortex (Martin del Campo et al., 2012). In the same line, several human studies have found brain structural changes in patients with hearing loss, including gray matter volume reduction in the right temporal lobe (Lin et al., 2014; Peelle and Wingfield, 2016) and correlations between hearing impairment and smaller gray matter volume in the auditory cortex (Peelle et al., 2011; Eckert et al., 2012). However, whether the atrophy of specific brain regions and cognitive domains in presbycusis patients are associated with cochlear receptor cell loss is unknown (Shen et al., 2018).
There are two types of receptor cells in the mammalian cochlea: inner hair cells (IHC) and outer hair cells (OHC). While, IHCs are related to sensory transduction of acoustic vibrations, OHCs are responsible for the cochlear amplifier mechanism, which allows high sensitivity and sharp frequency selectivity to low-intensity sounds (Brownell et al., 1985; Robles and Ruggero, 2001; Liberman et al., 2002). In natural hearing, the cochlear amplifier is fundamental for frequency discrimination and speech perception, and can be assessed through otoacoustic emissions (Oghalai, 2004).
Here we hypothesized that a cochlear amplifier dysfunction in presbycusis – an OHC loss – is associated with cognitive decline and structural brain changes in the elderly population. We studied the possible associations between cochlear OHC function (measured by distortion product otoacoustic emissions [DPOAE]), cognitive performance, and brain structure (magnetic resonance imaging, MRI) in a Chilean cohort of elders without dementia (ANDES) and with different levels of hearing loss.
Materials and Methods
All procedures were approved by the Ethics Committee of the Clinical Hospital of the University of Chile, permission number: OAIC752/15. All subjects gave written informed consent in accordance with the Declaration of Helsinki.
Subjects
For this cross-sectional prospective study, a total of 142 patients aged ≥ 65 years (screened between 2016 and 2018) from Recoleta’s primary health public center located in the city of Santiago (Chile) were evaluated for recruitment. According to the inclusion and exclusion criteria of the Auditory and Dementia study (ANDES), 96 subjects were included for neuropsychological, audiological and brain imaging assessments. Inclusion criteria were the following: patients older than 65 years without dementia, which was evaluated by a mini-mental state examination (MMSE) score ≥ 24 and preserved functionality measured by the Pfeffer activities questionnaire (Quiroga et al., 2004). Exclusion criteria of recruitment in the study were the following: (i) stroke or other neurological disorders; (ii) dementia; (iii) major psychiatric disorders; (iv) other causes of hearing loss different from presbycusis (e.g., conductive hearing loss); (v) patients using hearing aids and (vi) other causes of significant disability.
Audiological Evaluations
All the audiological evaluations were carried out by an experienced audiologist in a double wall soundproof room located in the Otolaryngology Department of the Clinical Hospital of the University of Chile. Air conduction pure tone audiometric (PTA) hearing thresholds were evaluated at 0.125, 0.25, 0.5, 1, 2, 3, 4, 6 and 8 kHz for each subject in both ears using a clinical audiometer (AC40, Interacoustics®). In addition, in order to rule out conductive hearing loss, bone conduction thresholds were measured at 0.25, 0.5, 1, 2, 3 and 4 kHz. All audiometric data presented in the present work refer to air conduction thresholds. The PTA at 0.5, 1, 2 and 4 kHz were calculated for each subject in both ears. Subjects were classified according to their hearing level: normal hearing (≤20 dB), mild presbycusis (>20 and ≤35 dB), and moderate presbycusis (>35 dB) based on the PTA of the better hearing ear. Moderate presbycusis subjects were referred to the Chilean program for hearing aids for elders >65 years.
Distortion Product Otoacoustic Emissions
Otoacoustic emissions are sounds emitted by the OHCs of the inner ear that can be measured through sensitive microphones (Kemp, 1978). DPOAEs are a type of otoacoustic emission, commonly measured in clinical and basic research as a non-invasive tool for assessing the OHC function of the cochlear receptor (Abdala and Visser-Dumont, 2001). In this study, DPOAE (2f1–f2) were measured as a proxy of the cochlear amplifier function, using an ER10C microphone (Etymotic Research®), presenting eight pairs of primary tones (f1 and f2, at 65 and 55 dB SPL, f2/f1 ratio of 1.22) in each ear at eight different 2f1–f2 frequencies: 707, 891, 1122, 1414, 1781, 2244, 2828 and 3563 Hz. Figure 1A shows an example of the spectrogram of the microphone signal obtained from the external ear canal of the subjects, in which a 2f1–f2 DPAOE peak can be observed. To consider the presence of a detectable DPOAE, we used an amplitude criterion: the amplitude of a given DPOAE (dB SPL) should be at least 6 dB above noise floor. Using this criterion, we counted the number of detectable DPOAEs per ear, going from 0 to 8: “0” means that the subject had no detectable DPOAE in that ear, and consequently that ear was classified as having cochlear amplifier dysfunction (see below), while “8” means that the subject had detectable DPOAEs at all tested frequencies in that ear (normal cochlear amplifier function); and “16” means that the subject had detectable DPOAE in all tested frequencies in both ears. In contrast with a method that only measures the amplitude of DPOAE, the procedure of counting the number of detectable DPOAE per ear allowed us to evaluate the cochlear amplifier function in the whole sample (n = 96), without eliminating subjects with no detectable DPOAEs (and no measurable amplitude in dB SPL), which is common in elderly subjects.
FIGURE 1
Figure 1. DPOAE measurements. (A) Example of the spectrogram of the microphone signal used to obtain the 2f1–f2 DPOAEs in response to f1 and f2 stimuli. DPOAE amplitudes are about 40–50 dB smaller than f1 and f2 primary tones. (B) Mean DPOAE amplitudes in response to the eight frequencies for left (blue) and right (red) ears (data shown as mean ± SEM). Notice that low frequency DPOAEs are larger in amplitude than high frequency DPOAEs. (C) Frequency histogram of the number of detectable DPOAE per subject for left (blue) and right (red) ears. Note that 24 and 26 subjects had no detectable DPOAEs in right and left ears, respectively.
Cognitive Assessment
All subjects and their relatives were evaluated by a neurologist during a structured interview and graded according to their cognitive complaints using the clinical dementia rating scale (Morris, 1993). Cognitive performances were assessed by an experienced psychologist in cognitive tests adapted for the Chilean population (Quiroga et al., 2004), including the MMSE for global cognition (Folstein et al., 1975), the Frontal Assessment Battery (FAB) for measuring executive function (Dubois et al., 2000), the Trail Making Test A (TMT A) for processing speed (Army Individual Test Battery, 1944), the Boston Nominating Test for Language (Kaplan, 1978), the Rey–Osterrieth Complex Figure Test for Visuospatial Abilities (Rey, 1959), the Backward Digit Span for Verbal Working Memory, and the total recall of the Free and Cued Selective Reminding Test (FCSRT) to explore verbal episodic memory (Grober et al., 1988). Depression was self-rated by the participants using the Geriatric Depression Scale (GDS), which consists of 15 YES/NO answer questions. Higher scores indicate higher levels of depression, with scores over five indicating significant depressive symptoms (Yesavage et al., 1983). Cognitive training was not performed in this study.
Magnetic Resonance Imaging
Neuroimaging data were acquired by a MAGNETOM Skyra 3-Tesla whole-body MRI Scanner (Siemens Healthcare GmbH®, Erlangen, Germany) equipped with a head volume coil. T1-weighted magnetization-prepared rapid gradient echo (T1-MPRAGE) axial images were collected, and parameters were as follows: time repetition (TR) = 2300 ms, time echo (TE) = 232 ms, matrix = 256 × 256, flip angle = 8°, 26 slices, and voxel size = 0.94 × 0.94 × 0.9 mm3. T2-weighted turbo spin echo (TSE) (4500 TR ms, 92 TE ms) and fluid attenuated inversion recovery (FLAIR) (8000 TR ms, 94 TE ms, 2500 TI ms) were also collected to inspect structural abnormalities. A total of 440 images were obtained during an acquisition time of 30 min per subject.
Morphometric Analyses
To determine the structural brain changes of controls and presbycusis individuals, we measured the volume and thickness of bilateral cortical regions. FreeSurfer (version 6.0)1 was used with a single Linux workstation using Centos 6.0 as was suggested by Gronenschild et al. (2012) for T1-weighted images analysis of individual subjects. The FreeSurfer processing involved several stages, as follows: volume registration with the Talairach atlas, bias field correction, initial volumetric labeling, non-linear alignment to the Talairach space, and final volume labeling. We used the “recon-all” function to generate automatic segmentations of cortical and subcortical regions. This command performs regional segmentation and processes gross regional volume in a conformed space (256 × 256 × 256 matrix, with coronal reslicing to 1 mm3 voxels). The function “recon-all” creates gross brain volume extents for larger-scale regions (i.e., total number of voxels per region): total gray and white matter, subcortical gray matter, brain mask volume, and estimated total intracranial volume.
Additionally, we measured the cortical thickness in native space using FreeSurfer tools. We calculated the cortical thickness of each mesh of vertices by measuring the distance between the point on one surface and the closest conforming point on the opposite surface. Then we measured the average of the two values calculated from each side to the other (Fischl and Dale, 2000). Based on the brain regions that have been previously studied in presbycusis (Lin et al., 2014; Rigters et al., 2017) our regions of interest were the bilateral frontal superior, temporal inferior, middle and superior gyri, parahippocampus, hippocampus, and amygdala. We also included as regions of interest, cortical areas that have been implicated in the neural networks of degraded speech comprehension: bilateral anterior cingulate cortex (ACC), posterior cingulate cortex (PCC), and precentral and postcentral gyri (Peelle and Wingfield, 2016).
Statistical Analysis
SPSS software version 20.0 was used to perform statistical analysis of demographic data, audiometric measurements, neuropsychological test results and structural MRI data. The ANCOVA method was used to compare cognitive performances and volume and thickness differences between the three tested groups including age, sex, and years of education as covariables. Pearson correlations were performed according to the distribution of data.
We applied general linear models (GLM) to contrast the difference of correlations between the studied groups using PTA as predictor for both volume and thickness. Age, education and sex were adjusted as covariates. The results were corrected for the multiple comparison using a significant alpha value of p = 0.01 (Hagler et al., 2006). Regions with significant differences in GLM models were selected based on the results of multiple corrections, and their corresponding brain regions were represented using the scientific notation p-values. The volume and thickness of selected regions were calculated based on the spatially normalized images of each individual in Talairach space (Fischl et al., 2002). In addition, we performed partial correlations including age, sex and education as confound regressors to associate cognitive tests with anterior and PCC volume and thickness.
Results
Demographics Characteristics
The mean age of the recruited subjects (n = 96, 63 female) was 73.6 ± 5.3 years [mean ± standard deviation (SD)] with an average educational level of 9.5 ± 4.2 years of schooling (mean ± SD). The audiometric hearing thresholds were normal in 32 subjects, while 44 and 20 individuals were considered as mild and moderate presbycusis, respectively. None of the 96 subjects used hearing aids at the moment of recruitment. A summary of demographic data, including age, sex, education, hearing level, smoking, and cardiovascular risk factors is presented in Table 1.
TABLE 1
Table 1. Demographic characteristics of the ANDES cohort.
Cochlear Amplifier Function
Figure 1B shows the mean amplitudes of the DPOAEs measured at eight different frequencies per ear, while Figure 1C displays a frequency histogram of the number of detectable DPOAE per ear, showing a bimodal distribution with a peak below two DPOAE per ear, and a broad distribution for more than two detectable DPOAE per ear. According to this bimodal distribution of the number of detectable DPOAE, we decided to split data into two groups: (i) better preserved cochlear amplifier function (≥5 DPOAE in both ears) and (ii) cochlear amplifier dysfunction (20 dB PTA, better hearing ear) with preserved cochlear amplifier (PCF) (n = 33); and (iii) mild and moderate presbycusis (>20 dB PTA, better hearing ear) with cochlear amplifier dysfunction (
Read Full Article (External Site)
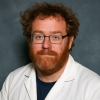
Dr. David Lowemann, M.Sc, Ph.D., is a co-founder of the Institute for the Future of Human Potential, where he leads the charge in pioneering Self-Enhancement Science for the Success of Society. With a keen interest in exploring the untapped potential of the human mind, Dr. Lowemann has dedicated his career to pushing the boundaries of human capabilities and understanding.
Armed with a Master of Science degree and a Ph.D. in his field, Dr. Lowemann has consistently been at the forefront of research and innovation, delving into ways to optimize human performance, cognition, and overall well-being. His work at the Institute revolves around a profound commitment to harnessing cutting-edge science and technology to help individuals lead more fulfilling and intelligent lives.
Dr. Lowemann’s influence extends to the educational platform BetterSmarter.me, where he shares his insights, findings, and personal development strategies with a broader audience. His ongoing mission is shaping the way we perceive and leverage the vast capacities of the human mind, offering invaluable contributions to society’s overall success and collective well-being.